From higher-resolution imaging to genome-sized DNA molecules built from scratch, the year ahead looks exciting for life-science technology.
Seven specialists forecast the developments that will push their fields forward in the year ahead.
SARAH TEICHMANN: Expand single-cell biology
Head of cellular genetics, Wellcome Trust Sanger Institute, Hinxton, UK.
In the past ten years, we have seen a huge increase in the number of single cells that researchers can profile at one time. That’s going to continue, mainly because of improvements in cell-capture technologies, methods that label cells with barcodes and smarter ways of combining existing technologies.
Such increases might sound mundane, but they allow us to do different kinds of experiment and to study more complex samples at higher resolution. Instead of just focusing on one person, for instance, researchers will be able to look at samples from 20 or 100 people at once. That means we’ll be able to get a better grip on population diversity. We’ll also be able to profile more developmental time points, tissues and individuals, enabling us to increase the statistical significance of our analyses.
Our lab has just participated in an effort that profiled 250,000 cells across 6 species, showing that the genes responsible for the innate immune response evolve rapidly and have high cell-to-cell variability across species — both features that help the immune system to produce an effective and fine-tuned response1.
We’re also going to see developments in our ability to look at different genome modalities at the same time in single cells. Rather than limiting ourselves to RNA, for instance, we’ll be able to see whether the protein–DNA complex called chromatin is open or closed. That has implications for understanding the epigenetic status of cells as they differentiate, and of epigenetic memory in the immune and nervous systems.
There’ll also be an evolution in methods of linking single-cell genomics to phenotype — for instance, in ways to link protein expression or morphology to a given cell’s transcriptome. I think we’ll see more of that type of thing in 2019, whether through pure sequencing or through imaging and sequencing combined. Indeed, we have been witnessing a kind of convergent evolution of both technologies: sequencing is gaining in resolution and imaging is becoming multiplexed.
JIN-SOO KIM: Improve gene editors
Director of the Center for Genome Engineering, Institute for Basic Science, and professor of chemistry, Seoul National University.
Protein engineering now drives genome engineering. The first-generation CRISPR gene-editing systems used the nuclease Cas9, an enzyme that cuts DNA at specific sites. This combination is still widely used, but many engineered CRISPR systems are replacing the naturally occurring nucleases with new variants, such as xCas9 and SpCas9-NG. These broaden the targeting space — the regions of the genome that can be edited. Some are also more specific than the first-generation enzymes, and that can minimize or avoid off-target effects.
Last year, researchers reported fresh obstacles hindering the introduction of CRISPR genome editing into clinics. These include activation of the p53 gene (which is linked to cancer risk); unexpected ‘on-target’ effects, such as large deletions; and immunogenicity against CRISPR systems2–4. To use genome editing for a clinical application, these limitations must be addressed. Some of these issues are caused by DNA double-strand breaks. But not all genome-editing enzymes create double-strand breaks — ‘base editors’ convert single DNA letters directly to another letter. As a result, base editing is both cleaner than conventional genome editing, and more efficient. Last year, researchers in Switzerland used base editors to correct a gene mutation in mice that causes phenylketonuria, a build-up of toxins5.
But base editors are limited in the sequences they can edit, which are defined by a sequence called a protospacer adjacent motif. Protein engineering can be used to redesign and improve current base editors and might even create new editors, such as a recombinase fused to inactivated Cas9. Like base editors, recombinases do not induce double-strand breaks, but can insert desired sequences at user-defined locations. RNA-guided recombinases are sure to expand genome editing in a new dimension.
The routine use of gene-editing techniques in the clinic might still be several years away. But we will see a new generation of tools in the next year or two: there are so many researchers interested in this technology, using it every day. Fresh problems crop up, but so, too, do innovative solutions. I expect to be surprised.
XIAOWEI ZHUANG: Boost microscopy resolution
Professor of chemistry and chemical biology, Harvard University, Cambridge, Massachusetts; and 2019 Breakthrough Prize winner.
The proof-of-principle demonstrations in super-resolution microscopy happened just a decade or two ago, but today the technique is relatively commonplace and accessible to biologists — and has led to a boom of knowledge.
One particularly exciting area of research is determining the 3D structure and organization of the genome. It is increasingly obvious that the genome’s 3D structure plays an important part in regulating gene expression.
In the past year, we have reported work in which we imaged chromatin (which forms chromosomes) with nanometre-scale precision, linking it to sequence information across thousands of cells of different types6. This spatial resolution was one to two orders of magnitude better than our previous work, and enabled us to observe that individual cells organize their chromatin into domains that vary greatly from cell to cell. We also provided evidence as to how those domains form, which leads us to a better understanding of the mechanisms of chromatin regulation.
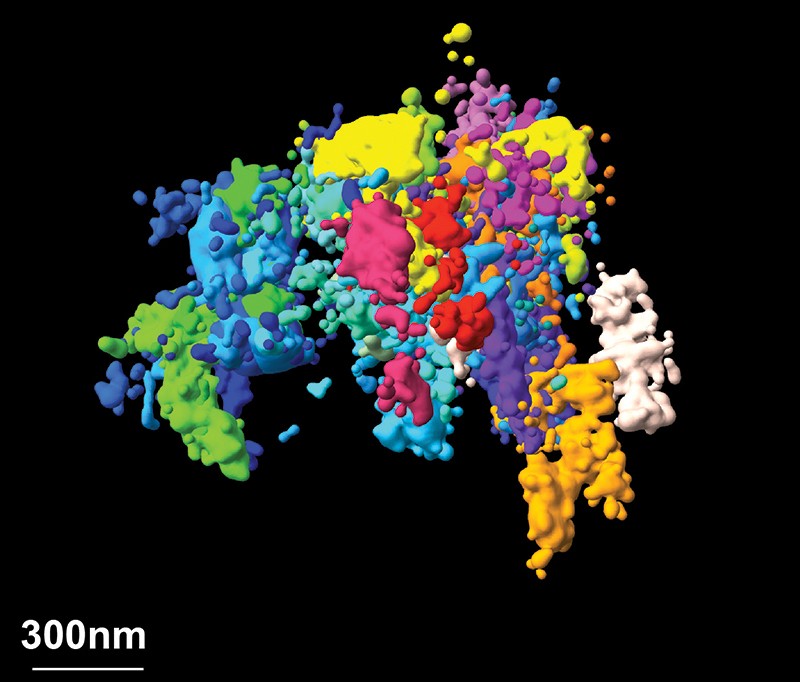
Beyond chromatin, I foresee substantial improvements in spatial resolution across the field of super-resolution imaging. Most experiments operate at a resolution of a few tens of nanometres — small, but not compared with the molecules being imaged, particularly when we want to resolve molecular interactions. But we are seeing improvements in fluorescent molecules and imaging approaches that substantially increase resolution, and I expect that imaging at 1-nanometre resolution will become routine....MUCH MORE
At the same time, temporal resolution is getting better and better. At the moment, researchers must compromise between spatial resolution and imaging speed. But with better illumination strategies and faster image acquisition, those limitations could be overcome. Tens of thousands of genes and other types of molecule function collectively to shape a cell’s behaviour. Being able to watch these molecules in action simultaneously at the genomic scale will create powerful opportunities for imaging.
HONGKUI ZENG: Map brain connections
Executive director of structured science, Allen Institute for Brain Science, Seattle, Washington.
The connections between individual cells and various cell types are so complex that mapping their connectivity at the global and population level is no longer sufficient to understand them. So, we are mapping connections based on cell type and at the single-cell level.
We can accomplish this with ‘anterograde’ and ‘retrograde’ tracing,...